This post is prompted by recent posts by Steve Goddard on WUWT about the GHE and the lapse rate on Venus. They muddle the effects, in a way that is quite often seen in the blogosphere. The meme is that surface warming is due to the lapse rate and not to the GHE. Often on WUWT this comes down to even more simplified assertions that warming is due to atmospheric pressure.
The fact is that the dry adiabatic lapse rate, and the mechanism that creates it, are an intrinsic part of the greenhouse effect that causes warming at the surface. More below the jump.
The elementary GHE explanation
A brief explanation of the GHE usually notes that the atmosphere is differentially transparent, letting most sunlight through, but obstructing IR. This is described as trapping heat, or blanketting or whatever.
This sometimes is criticised for inexactness. It's true that the heat isn't trapped - it all gets out. And a blanket isn't quite right.
Still, the reasoning does reliably lead to the right answer. With physical situations, where there is a flow driven by siome kind of potential and the flow is impeded in some way. the potential builds up behind the impedance. It has to, in order for the flow to get through. It happens with electrical circuits, river flows, even in economics. But it's true that more can be said about the mechanism.
The dry adiabatic lapse rate
I posted a while ago on the dry adiabat and the atmospheric heat pump which maintains it. Any gas in motion in a gravity field will have a vertical component to the motion. When it moves down or up, it is compressed or rarefied. Respectively, it is heated or cooled. This heat change then diffuses in to the gas at the new level. Both up and down motions have the effect of pumping heat downward, until the dry adiabat lapse rate is achieved. This is a temperature gradient, warming in the downward direction, and the critical level is g/cp, where g is the acceleration due to gravity and cp is the constant pressure specific heat of the gas.
As I said, maintaining such a gradient requires a heat pump, because heat tends to move down the gradient by conduction. The energy for the pump comes from atmospheric motions, which are thus attenuated. But when a large heat flux is passing through the air, as in the solar flux which causes all kinds of differential heating, there is energy to drive and sustain these motions. And because the energy sink is the need to overcome conductive leakages, the drain is small, because, the conductivity is low (although there are other demands too).
Adiabat, solar flux and no GHG
Suppose then we have an atmosphere of nitrogen, which does not absorb or emit IR. Sunlight is converted to heat at the surface, and to maintain energy balance, this heat must be radiated back to space.This goes straight through the N2. The surface will warm to just the temperatrure that is needed, on average, to achieve that outward radiation level.
The surface will not be uniform. Some parts will be hotter than others, and this will set up local convection cells. Bigger cells will take heat from the tropics to colder parts. The N2 will be in motion.
So, I hear you say, shouldn't the energy balance include convection from the surface. Well, there will be some exchanges, but on balance, heat flux to the N2 goes nowhere. For N2 cannot emit it to space. It can only conduct it back to the surface (somewhere).
The important thing to note is that the dry adiabat has nothing to do with IR properties. It only requires gravity and motion. The N2 atmosphere will have that g/cp gradient. But it will start from the surface temperature fixed by the IR balance, and get colder as you go up.
Snowball Earth
An example often cited is of the Earth without GHG's. To keep in balance with sunlight, after allowing for albedo, the Earth has to emit about 235 W/m2. At a uniform surface, the temperature required to do this is about 255K. This is much less than the temperatures we have, and the difference of about 33K is ascribed to the greenhouse effect.
Greenhouse gases
When there are GHG's that absorb and emit IR, there are many changes. One thing that doesn't change, though, is that the heat absorbed from the Sun must still get out to space. The question is what warming will occur to make that happen.
One thing that does change is what actually emits the heat. GHG's, like surfaces, emit according to their temperature. At many wavelengths, the main GHG's, H2O and CO2, are dense enough to absorb nearly all the IR emitted from the surface. Kirchhoff's Law says that, at each wavelength, emissivity equals absorptivity. So at these absorbing wavelengths, water and CO2 are also the main sources of emission that go out to space. At other wavelengths (the atmospheric window), the emission to space is direct from the ground.
But the absorption and emission happen at different places. Much of the IR is absorbed soon after leaving the ground. At those frequencies, IR is also emitted from the same gases high in the atmosphere. The absorption and emission are not directly connected. The heat absorbed has to somehow reach those upper levels before emission can occur. That is another interesting story.
The adiabat and heat balance
Meanwhile, the adiabat is still there. It is determined by the properties of the non-GHG gases (at least on Earth). And it ensures that the emission from GHG occurs at a much lower temperature than the surface.
For the adiabat, I've used the analogy of a battery, which maintains a voltage difference between its ends of, say, 1.5V, but does not determine the actual voltage there. That depends on how it is "earthed". In the non-GHG case, it is "earthed" at the ground, where the temperature is fixed by radiation balance. Since no "current" (heat flux) flows through the battery at the top, it's voltage is just 1.5V less, passively determined.
When emission occurs from GHG's at TOA, a similar heat balance equation determines the temperature there. That is where the adiabat "battery" is "earthed". The temperature gradient below is still fixed, and hence so is the surface temperature - at a much higher level. That is the complete greenhouse effect.
So for the Earth?
If GHG's had uniform strong effect over IR frequencies, then the whole 235 W/m2 would be emitted from TOA, and this would have to happen at the snowball earth temperature of 255K, on average. TOA for this purpose is probably about 10 km or more, so the Earth's surface would be about 355K. very hot. The actual location of the emission depends on the GHG concentration. If it is lowered, some of the emission will come from lower levels, reducing the average height, and hence the temperature at the surface.
It should be said too, that on Earth the dry adiabat is rarely attained. That is mainly because the air is not dry, and water phase changes reduce the gradient considerably.
In fact, as I've mentioned, the Earth has an atmospheric window, through which some of the IR (about 40 W/m2) is emitted with a spectrum corresponding to the warmer ground surface. That leaves less to be emitted at the top, and so the temperature can be lower. Indeed, the effective emission from GHG's is at about 225K - so instead of a uniform 255K emission temp, we have part at about 288K and part at about 225K. That reduces the ground temp by about 30K.
Spectra
You can see how this works for the Earth from observed spectra. Below is a plot of IR spectra observed near Barrow, Alaska (source , from Grant Petty's textbook "A First Course in Atmospheric Radiation"). The top plot shows , in effect, outgoing IR. It's only part of the thermal spectrum, but shows a dip between 600 and 800 cm^-1, which is emission from CO2, and to the right of that, the atmospheric window, where IR comes largely unimpeded from the surface. Note that in the dip it tracks the BB curve for about 225K, and in the window, about 268K - the spectrum is taken over a polar ice sheet near Barrow AK. The lower plot shows the IR reaching the surface below. Corresponding to the emission CO2 dip above, there is a peak caused by atmospheric (low level) CO2 emission.
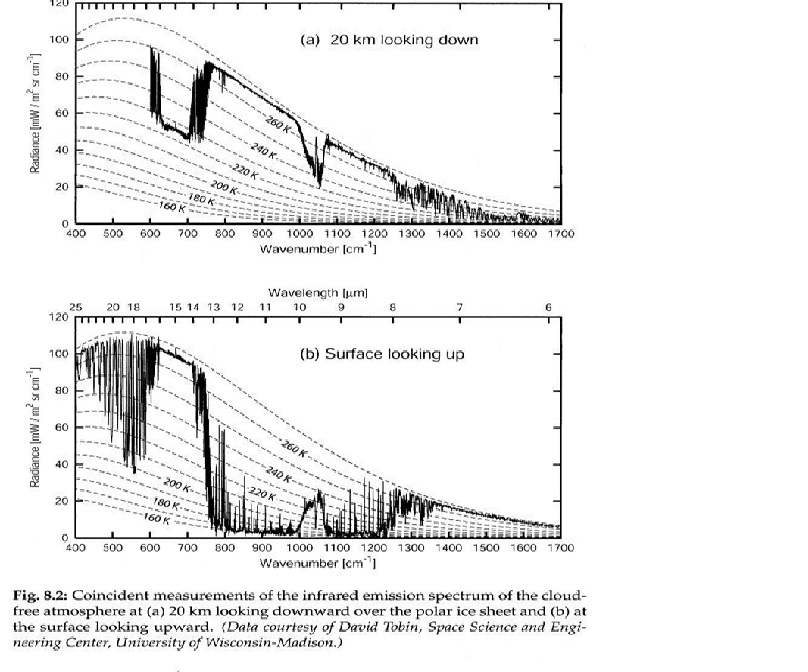
It might be worth adding a discussion of pressure broadening in the spectra section.
ReplyDeleteThe Greenhouse Effect (GHE) and the lapse rate.
ReplyDeleteThe phrase GHE muddles together the effect and the suggested cause. It suggests that the warming effect of the atmosphere is caused by the chemical structure of some of the gases in air, molecules that are made of more than one type of atom, especially CO2.
We all agree that there is a warming in addition to that caused by the sun. What causes the extra warming?
Steve Goddard’s post at WUWT says the extra warming is explained by pressure of the atmosphere. There is no need to consider the chemical composition. All gases create pressure. All gases contribute to the extra warming. All of the extra warming is explained by physics. There is no need to consider the structure of these gases.
Zeke,
ReplyDeleteI'm mainly trying here to clarify the relation between GHG behaviour and the lapse rate in the GHE. But yes, pressure broadening was obviously very relevant to the WUWT Venus example particularly.
Roger,
ReplyDeleteI wouldn't exactly agree that there is warming extra to that caused by the sun. There's no extra energy. It's more like an insulated house, where you get a higher temperature for the same heat input.
It's Steve's pressure theory that I'm disputing here. The gas compressibility and gravity pressure gradient create a temperature differential. That doesn't in itself warm the surface, and without GHG the surface would be cool, and the gas temp would just get even cooler as you go up, thus maintaining the gradient. But with GHG's, you are fixing the temp at a higher altitude (TOA), and then the temp diff forced by the compressibility comes into play, ensuring that the surface is much warmer.
Eli thinks this is too complicated. It's called the dry adiabatic lapse rate, which means that it is an idealization where the energy flow into and out of any packet of air is balanced wrt condensation of water vapor, radiation and convection.
ReplyDeleteThe surface temperature is fixed by a radiation balance between incoming solar and out and incoming IR and the TOA is at 3K or whatever near space is.
That leaves gas rarification driven by gravitation as the mechanism for setting the atmospheric temperature profile
Except it's not the dry adiabatic lapse rate (~ 10°K/km), it's the environmental lapse rate (~6.5°K/km). Picking nits admittedly, you said "dry lapse rate", water vapor doesn't play a role.
ReplyDeleteAnd of course the lapse rate is only approximately maintained from the top of the atmospheric boundary layer to the tropopause. Above there, the temperature begins to increase due to absorption of solar UV radiation by ozone gas.
Finally 3*K has nothing to do with "the top of atmosphere" as far as radiative equilibrium is concerned. That height is the height at which the air becomes rarified enough to neglect further gases above that height, which you can read from Nick's plot is around 220°K (not coincidently the temperature at the tropopause).
Eli
ReplyDelete"where the energy flow into and out of any packet of air is balanced wrt condensation of water vapor, radiation and convection"
This was a puzzle to me - I don't think it is right. Obviously not condensation, ("dry"?). Nor convection - the packet of air is assumed to stay together, so nothing can convect out. Finally radiation? No, the theory applies to all gases, not just those with a GHG component.
The dry lapse rate is just the gradient at which cooling by rarefaction of rising gas happens at just the same rate as the ambient temp changes.
And I agree with Carrick - the TOA layer (about at tropopause) is where you have a radiative balance between IR out (to the 3K space) and SW absorbed (mostly further down). That sets the temp to about 225K, not 3K.
But I think I agree with the last sentence, if you say rarefaction and compression, and add the requirement for forced motion. Maintaining a lapse rate actually drains energy from something, otherwise conduntion would smooth it out.
Nick,
ReplyDeleteIt's ADIABATIC, that means no net heat flow (strictly speaking, no heat flow at all) so all the mechanisms of heat flow, radiation, convection, latent heat, etc. can be ignored for the purpose of calculating the dry adiabatic lapse rate, and all you are left with is the gravitational compression/rarification.
You get the same result (and believe it or not, G&T published this on arXiv as an important discovery), if you start with Navier-Stokes and set the vertical transport to zero everywhere.
The reason for rarification is that it better expresses the reason for observing a cooling with altitude, but compression/rarification also works depending on which end you start at.
Carrick, the idea collapses at 220K for the Earth's atmosphere because you have an ozone layer, e.g. the system is no longer adiabatic. OTOH, if you had a pure N2 atmosphere, the lapse rate would continue to the top
ReplyDeleteEli, we don't have a pure N2 atmosphere and if we did there wouldn't be much of a GHG effect. Anyway, even for a N2 atmosphere, at high enough elevations, you would still have a thermosphere (and this would happen even in a pure N2 atmosphere).
ReplyDeleteThe point of this is that any description of the actual mechanism for GHGs (as opposed to a "sound bite") can't depend on a lapse rate surviving to the 3K boundary.
Fortunately it doesn't have to as both Nick and I've pointed out. The lapse rate merely needs to make it to the altitude where radiation balance is achieved.
And btw, the atmospheric processes that create a net 6.5K/km lapse rate are not entirely adiabatic. Radiative and advective transport of heat in the atmosphere are generally described as non-adiabatic. If one does the calculation as you suggest, you get an answer that is off by 35%.
There's collisionally induced absorption/emission in the thermal IR even for N2, not much, but not negligible for an N2 only atmosphere. I propose the invention of a new gas, unobtaineon, Ub (closely related to the metallic element unobtanium, Un, also with magical properties), that is monatomic, ideal at all temperatures and perfectly transparent at all wavelengths for illustration purposes. I haven't decided on an atomic weight yet. Maybe 29 so it matches the average atomic weight of the Earth's atmosphere.
ReplyDeleteAnyway, a pure Ub atmosphere cannot have positive lapse rate (meteorologic convention) everywhere. For heat to be transferred to the surface from the atmosphere by conduction, the lapse rate must be negative. The average lapse rate for the planet as a whole must be close to zero for heat transfer to balance.
This pressure causes temperature meme is really annoying. The surface pressure is fixed by the mass of the atmosphere, presumably a constant. The atmosphere can be any temperature so temperature is the independent variable with volume as the dependent variable.
DeWitt,
ReplyDeleteI think scale is the issue here. Tle lapse rate is small on the scale of boundary layer turbulence. The region close to the ground where heat is conducted is very small, and may have a high gradient in any direction. Once above that layer, with turbulence you get the heat pump and the adiabat.
But your Ub atmosphere is neither a heat source or sink, Nett flow to the ground should be nil.
I still think there would be motion, a heat pump, and an adiabat. Just no nett average heat flow through any layer of the Ub.
There's one feature of Ub that I like - ideal gas at all temperatures. One fly in the ointment with a massive N2 atmosphere on Venus is that with a lapse rate, fairly cool surface and no IR effect producing a tropopause, is that N2 would liquefy at high altitudes. I'm not sure how that would work.
Nick,
ReplyDeleteA heat pump requires an energy source that can supply enough power to operate it. I remain unconvinced that conduction from the surface has the capacity you need. You not only need enough power to do the heavy lifting, but also enough power to overcome frictional and other dissipative losses for the horizontal transport of energy.
Your description of physics of GHE is unexpectedly correct, with exception of few details.
ReplyDeleteFirst, you state, “Any gas in motion in a gravity field will have a vertical component to the motion.” This is a frequent misconception and is generally not true. The thing is that any gas has molecular viscosity, and without continuous source of stirring all motion fill fade away and eventually come to total halt. If the atmosphere is fully spherically symmetrical (which means that the surface is heated uniformly), the macroscopic motion will stop, and the optically-inert atmosphere will become isothermal. Luckily for us, it is really hard to make the surface heated uniformly given a point-like source of energy like Sun, so this never happen, and we enjoy side effects of [wet-]adiabatic lapse rate. And planet’s rotation does help too.
Second, you assert, “But the absorption and emission happen at different places. … The absorption and emission are not directly connected.” This statement will severely offend baseline physicists, because absorption and emission ARE directly connected: at a given temperature of an object, in thermodynamic equilibrium, whichever gets absorbed is emitted right away. What you are referring to is a compound concept of gas opacity in certain radiation bands, intertwined with the concept of LTE (Local Thermodynamic Equilibrium), and diffusion approximation of radiation fluxes. Yes, it is true that certain wavelengths (near 14-16um, or 600-700 cm-1) get absorbed in less than 1 meter of path, but this layer would also emit nearly all this back, which is called “back radiation”. In the middle of troposphere every air parcel absorbs and instantly emits in accord with local balance, such that only a weak gradient of flux exists. You are right that the main transfer of heat occurs by macroscopic movement of air, which transports the major heat from surface layers up into “effective emission layer”. Interestingly, this layer has almost nothing to do with tropopause or other mechanical definition of TOA (Top of Atmosphere). The TOA is not where gas gets rarified, it is where it loses its optical opacity.
Contrary to beliefs of some scientists from unrelated fields, the lapse rate will never continue “to the top”. The lapse rate will continue only to the point of gas density which is able to support convective instability. The entire lapse “adiabatic [polytropic] engine” is driven by buoyant instability resulted from gas being heated from below, from ground surface. This instability provides the mixing. But as density of gas diminishes with height, the corresponding governing number (Reynolds/Rayleigh, or whatever) gets below critical value, and buoyant convection is no longer sustainable. Inversion of temperature profile above tropopause obviously plays in the same direction.
Now, once you spelled out the true physical mechanism of GHE, it will be interesting to hear your opinion about sensitivity of this effect to particular changes in CO2. This might be very illuminating.
Well, unexpected agreement all around :) Mostly.
ReplyDeletevertical component This is just straight fluid mechanics. If you have just horizontal motion, there must be a vertical velocity gradient, since the velocity is zero next to the ground. That means all kinds of vorticity roll-up etc. It's virtually impossible to maintain 2D motioneven in the lab, where you often would like it.
the absorption and emission happen at different places By the absorption I mean the first absorption of IR emitted from the ground, and emission is final emi9ssion at TOA. Hence the different places. Of course, the emitted heat ultimately has to come (mostly) from the heat absorbed, which requires transport, and much of that is by re-emission.
But I agree with your mention of LTE. People often think that IR is emitted from the same molecule that absorbed it. But no, LTE says that the KE from absorption is shared among all nearby molecules (GHG and not), and the energy for emission comes from neighboring molecules too. You say what gets absorbed gets emitted right away, but that's just an expression of steady state, not a mechanism. It would be quite possible to have more emitted than absorbed - the air would just cool. Generally it doesn't.
And indeed, TOA is the end of opacity. I'd like to claim credit for being right about most heat being transported by air movement, but that isn't what I believe. Most is transported by radiation, merging into the Rosseland regime, air to air down a temperature gradient. The adiabatic heat pump is important in maintaining the temperature gradient, but doesn't transport much heat.
I agree that the lapse rate can't continue to the top, but not for reasons of convective stability. The unstable region is where the temp grad is steeper than the adiabat; the adiabat is neutral, and for gradients between that and isothermal (or below) convection is increasingly heavily damped. The adiabat is independent of density, so that doesn't change as you go up.
What brings the adiabat to an end is the end of opacity that you referred to. At that point there is nett emission to space, and the energy for that flows in from above and below. The emission does not have an associated temp gradient, but the fluxes to supply it flow generally down a temp gradient. So the emission sink must be a temperature minimum.
At the tropopause the gradient goes through zero; much convective damping. That's why the tropopause is a barrier to vertical transport, and the stratosphere has no weather.
You mention "adiabatic engine". I argued a while ago that it's an engine only when the temp gradient exceeds the dry adiabat. Below that, it's a pump, driven by winds, which in turn are driven by thermal inhomogeneity (an engine).
So OK, sensitivity to CO2? This really comes from height of TOA. With more CO2, the region where there is clear emission to space is higher. The temperature of this region is fairly fixed (S-B), because the flux that has to be emitted is determined by insolation. So if the emission region is higher, the lapse rate ensures that the ground is warmer.
Why do you believe that emission changes much with change in CO2? What goes up is the AVERAGE TOA. However, the emission-absorption spectrum of CO2 is highly uneven, and total _change_ in flux from changes in TOA for individual lines is not so obvious.
ReplyDeleteConsider an example. Let one line (or a narrow band, like 14-16um) have strong absorption, and another wider area (say, 2x) with very weak absorption. Their average “emission height” is, say, at 5-6km. According to the standard theory of averages, the ”average emission height” will go up with increase of CO2, where "higher= colder". Colder layer emits less, and therefore the global imbalance of OLR would occur forcing climate to warm up.
However under a more careful view, this average of 5-6km is made of 0km emission height for 2/3 of IR range, and 18 km emission height for the remaining 1/3. Increase of 0 km band gives you zero change in OLR, while increase in 18km emission height will give you MORE OLR, because the temperature gradient in stratosphere is opposite to one in troposphere, so “higher = warmer”. As result, the warmer layer would emit more, and the energy imbalance would be POSITIVE implying COOLING, or just exactly opposite to what the standard "averaging" theory says.
In reality the spectrum is more complex, many different trends coexist, and seems very likely to cancel each other in the first approximation. Therefore, the effect of CO2 increase is a second-order effect, and must be much harder to calculate. I know, I know, climate experts like Myhre and his school have calculated the fluxes directly. However, they assert that the entire "forcing" can be calculated from only three atmospheric profiles, tropical and two extratropical. I find it hard to believe that polar areas play no role in global radiative balance of the planet.
"What goes up is the AVERAGE TOA."
ReplyDeleteYes, that's just what I said. "This really comes from height of TOA. With more CO2, the region where there is clear emission to space is higher. ".
I agree with you about the spectral variability. though I think the fraction emanating from 0km is closer to 1/3 (and there's another region in between).
The driver for meridional circulation is the pressure gradient force. At any given altitude above the surface, the pressure is higher for a warmer surface than a colder surface. The difference increases with altitude. But the Coriolis effect causes the flow from equator to pole to bend away from the poles. The end result is the formation of the jet streams in each hemisphere. The jet streams act to separate the cold polar air mass from the warm equatorial mass. So there is flow of heat from the equator to the poles, but not nearly as much as one might expect from the temperature difference alone.
ReplyDeleteThere's a good explanation starting here:
http://www.mit.edu/~predawn/jetstream/temp_grad.html
Nick, the "zero km" layer is formed from spectral area with zero absorption. Increase of concentration in area with zero absorption results in zero change. I would guess that a great deal of change in the "region in between" would fell into the height of tropopause, which is isothermal, and therefore any change in "emission height" would also result in no change in emission. The remaining narrow tails of Lorentzians likely to contribute to warming, it is true. So, no matter how to slice it, detailed LBL physics seems to suggest that there is at least cancellation of increases and decreases. The effect seems to be zero, in first approximation.
ReplyDeleteDeWitt, these explanations are all right, nice, correct, and handy. However, the problem at hand requires a pretty accurate quantitative answer. Unfortunately, the climate problem entirely is hanging on hydrodynamics of a very large system, with very complicated boundary conditions, with enormous value of Reynolds/Rayleigh number, where no solution can be obtained, not even numerical. It is very definitely that primitive equations like hydrostatic or other primitive models do not cut it. As an illustration of basic concepts, these models are fine, but they cannot solve the applied problem of climate stability and change.
ReplyDeleteAl Tekhasski,
ReplyDeleteMy comment has to do with a disagreement between Nick and I about the structure of a non-radiative atmosphere. I never suggested that it "solve[s] the applied problem of climate stability and change." But yes, our disagreement could probably be solved if either of us had access to a three dimensional general circulation model.
DeWitt,
ReplyDeleteThe Coriolis effect means that part of the heat engine is used to create jet stream KE. I'm, not sure how that affects the issue - jet stream turbulence also goes toward maintaining the lapse rate. It's true that it reduces the KE delivered to the primary circulating flow somewhat. But plenty remains.
Earlier Nick wrote, "Most is transported by radiation, merging into the Rosseland regime, air to air down a temperature gradient. The adiabatic heat pump is important in maintaining the temperature gradient, but doesn't transport much heat."
ReplyDeleteI am afraid you are mistaken here. True, the adiabatic process does not exchange much heat with surroundings, but the corresponding convective overturning carries out a lot of heat. If we consider the Kiehl and Trenberth estimations, radiation part of heat flux is 39% of total surface flux. Contrary to your statement (as implied by your reference to temperature gradient), the molecular diffusion transport is totally negligible. If you consider that the average flux of 240W/m2 happens across the air of 6km, the effective "thermal conductivity" of this layer appears as 500 times higher than thermal conductivity of copper, which is clearly not the case with air. From K&T budget, the evaporation-condensation heat pipe provides 50% of total heat flux, and remaining 11% is provided by pure advective-convective heat transport. Both pipes require mechanical overturning. Unfortunately, GCM do not model vertical transport from first principles of hydrodynamics. The models incorporate parameterized transports in accord with understanding of their creators (which is open to questioning), so they cannot help.
Al, "as implied by your reference to temperature gradient"
ReplyDeleteNo, the reference does not imply molecular diffusion, which is indeed negligible. Turbulent diffusive transport also varies with temperature gradient, and is larger. But I was referring to air-to-air radiation transport. People often don't have a clear idea of how this works, but it is also dependent on temperature gradient, and at the Rosseland limit (high opacity), is explicitly proportional.
Nick,
ReplyDeleteWhat happens if we increase the surface pressure of the Ub atmosphere to 100 bar? It looks to me like your model of dry adiabatic lapse rate everywhere all the time would imply an increase in surface temperature compared to a 1 bar atmosphere. But for me, the increase in thermal conductivity at the higher density would make an isothermal atmosphere even more likely.
But lets make it even more Venus-like. Put a cloud layer at the top of the atmosphere that's reflective in the visible and UV and absorbs completely everywhere else. The rest of the atmosphere is, of course, completely transparent. If we put this planet at the orbit of Venus and specify a that 76% of incoming radiation is reflected, then the brightness temperature of the cloud layer is 230 K. The surface sees 158 W/m2 from the cloud layer so it also has a temperature of 230 K and radiates 158 W/m2 back to the cloud layer and the atmosphere is isothermal and the energy flows balance in one dimension. Extend that to three dimensions and there will be energy flows, but the surface temperature and the cloud layer temperature will still be nearly the same at every point.
Now let's remove the cloud layer and move the planet further away from the sun so the energy absorbed by the surface is still 158 W/m2 on average. According to you, the atmosphere should revert to the adiabatic lapse rate. That would require the surface to emit more than it absorbs until all that kinetic and gravitational potential energy was radiated away. But where's the driving force for that to happen?
DeWitt,
ReplyDelete"What happens if we increase the surface pressure of the Ub atmosphere to 100 bar?"
It increases the temperature differential, top to bottom. The effect on surface temp depends on where the temp profile is "anchored".
With the cloud layer, it wasn't clear whether the remaining 24% light gets through or not. If it does, then it's a bit like the steel greenhouse. If the cloud is opague but conductive, the upflux reaching it must be 158*2 W/m2 and the surface temp is 230*2^0.25. If not very conductive, the temp on the bottom surface is higher, and the ground can be hotter.
The adiabatic lapse rate is over-ridden in this case (although there is a temp gradient), because the IR specifices the temp at two places (cloud and ground), and the IR exchange if the spec is not complied with exceeds the flux that the adiabatic heat pump can provide.
If you remove the cloud layer then yes, the surface will cool. Driving force? It's just like you cool in bed when the blankets are removed. The 158 W/m2 from the sun remains, but the 158 W/m2 back radiation is lost.
Nick, I noticed my misinterpretation. I am not familiar with original work of Rosseland in astrophysics (1931), but it sounds like the standard diffusion approximation widely used in 60-th in engineering works on industrial ovens and NASA studies
ReplyDeletehttp://ntrs.nasa.gov/archive/nasa/casi.ntrs.nasa.gov/19640018437_1964018437.pdf
, and likely was independently derived in studies of nuclear explosions, but for obvious reasons known to much lesser degree. In your reference to CFD user manuals, they mention the
diffusion equation q = -(k + kr)grad(T).
Do you happen to know the relative values of k and kr? It would help to understand the amount of contribution of radiation diffusion to overall heat flux. To my knowledge, when a totally opaque liquid is studied, radiative diffusion is always neglected, so it must give us some perspective.
Yes, it is - I first knew it as a P0 approx.
ReplyDeleteYou can get a feel for kr this way (k is negligible). The formula is, ignoring scattering, phase coefficient, refractive index effects etc:
F/F0 = (16/3a) (grad T)/T
where F0 is blackbody flux at T, F is nett transport, a is absorption coefficient. At the adiabatic lapse rate, grad T / T is about 0.04 km-1, so F/F0 ~ 0.2/a.
So if the characteristic length for absorption is 1 km, say, then the flux is about a fifth of BB flux. That's quite large. The argument can be subdivided into wavelength bands.
DeWitt wrote:
ReplyDelete"What happens if we increase the surface pressure of the Ub atmosphere to 100 bar? It looks to me like your model of dry adiabatic lapse rate everywhere all the time would imply an increase in surface temperature compared to a 1 bar atmosphere. But for me, the increase in thermal conductivity at the higher density would make an isothermal atmosphere even more likely."
Thermal conductivity of air does not change with pressure. According to CRC "Nandbook of Chemistry and Physics" 64-ed (that I happen to have on my bookshelf), this parameter is exactly the same at 1atm, or at 20atm, or at 40atm. Even with temperature variation of +-100K it does not change more than 25%. The specific heat at constant pressure stays the same too. Therefore the adiabatic process will be the same. As long as surface will continue to absorb incoming SW radiation and cause initial warm up, rapidly developing instabilities will stir the air, and convective mixing (and associated lapse rate) will take place. Coriolis-like instabilities will also contribute to air stirring and set the lapse rate even if optical properties will prevent SW from reaching the surface.
To get 100bar at surface at given g, the atmosphere must be 5x thicker as compared to today's. Therefore, the effective emission layer will be also about 5x higher, and GH effect must be 5x33k = about 150K. With a given solar constant and same albedo (and corresponding average emission temperature of 255K), this atmosphere will produce surface temperatures of about 400K.
This begs an interesting (to me) question: since the global temperature depends so much on total mass of atmosphere, is there any [geological] evidence that Earth atmosphere always had this particular mass? If the mass was bigger in the past for one or another reason, then there would be no need to invoke CO2 argument to offset the faint Sun of the past.
Al Tekhasski,
ReplyDeleteSorry I mis-stated. I meant thermal diffusivity not conductivity. The thermal diffusivity is a function of density. Worse, though is that I got the direction of the effect backwards. The thermal diffusivity decreases with increasing density so the rate of heat transfer by diffusion would actually be slower at higher pressure. In that respect it's similar to opacity and radiative diffusivity.
I'm beginning to see why Nick likes the Rosseland heat transfer model. For the Earth, it's only really applicable to narrow bands like the 667 cm-1 band for CO2, but it should work well under Venusian conditions.
Nick,
ReplyDeleteI've asked Gavin Schmidt about the lapse rate in a transparent atmosphere. I probably mis-stated your position and wasn't all that clear on mine. I think he thinks we're both wrong to some extent, but I'm probably more wrong than you. I would rather not post the email, but I can forward you a copy. Let me know where to send it.
After some thought and re-reading let me see if I have a better understanding of your heat pump. What I think you propose is that if you start with an isothermal atmosphere an constant illumination any horizontal air movement near the surface will generate a horizontal vortex with air going around a circle up and down. The air going up cools and absorbs heat from the warmer air above. It then moves downward, is heated by compression and transfers heat to the surface which is then radiated away. The end result is the creation of a temperature gradient. The process reaches steady state when the gradient reaches the adiabatic rate. Is that a correct interpretation? It looks very much like some of the slides in this Powerpoint presentation: lightning.sbs.ohio-state.edu/geo622/ch2_structure.ppt
I see at least one potential problem. It's probably true in the boundary layer, but above the boundary layer in the free atmosphere you don't have the surface drag and so the vertical component could be a lot smaller.
DeWitt,
ReplyDeleteYes, I'd be very interested to read what Gavin has to say. My email details are here or via the contact link on the right (under Resources).
On Rosseland, the reason I like it is not so much that it can be used quantitatively, but that it gives some basis for estimating how IR interacts with the gas temperature gradient. As you say, it's good for 667 cm-1, say, but then the flux is small. At an absorption length of 1 km, it's still OK, and the flux is moderate. As the length increases, the flux increases, but starts to interact less with the air temp grad, and in the AW, max flux but no interaction.
On the heat pump, I'm mainly thinking in terms of turbulence, rather than big structured eddies like Hadley cells. There's air going up and down, and in each direction it pumps heat down, for lapse rate below the adiabat.
But this is just one heat flux in the system. I liked the way you put it at SoD. If the temp grad needed to transpost the flux by IR etc (thinking Rosseland) exceeds the dry adiabat, then convection is generated, which carries the rest of the heat and brings the lapse rate down to adiabat. I'm putting a flip side - if the temp grad carrying the flux is less than the adiabat, then turbulence will drive the grad up to the adiabat level, if it can. This takes energy from the turbulence, so if the energy available is inadequate, the lapse rate will be somewhat less, as it often is on Earth.
I didn't see anything in the slide show that corresponds, although my Linux system doesn't show it perfectly. He's concerned with a 1km or so surface boundary layer, which is a different scale. He does talk about entrainment, but that's different, I think.
DeWitt,
ReplyDeleteThanks for sending Gavin's email. I'm very impressed by the care that Gavin took over the query. More for me to think about.
Nick,
ReplyDeleteI found this thread at Climate Change. I haven't worked my way through all the comments, but there are several that address the point of lapse rate in a transparent atmosphere.
What happened to the "cooling of the stratosphere"? The greenhouse hypothesis predicts an isothermal atmosphere in the absence of greenhouse gases.
ReplyDeleteAnders,
ReplyDeleteThe greenhouse hypothesis predicts an isothermal atmosphere in the absence of greenhouse gases.
I don't think that is true. I posted here about that.
Nick,
ReplyDeleteI just read your writeup, and completely agree with basically all you said. This is in fact what I thought I said. If you disagree, please tell me where. The difference we seem to have beyond that relates to my comments on the model I used with no Solar radiation being absorbed except at the blocking shell. The variations in temperature at different locations and different times would (I am sure) be enough to generate convective currents strong enough to drive a nearly full adiabatic lapse rate. You seem to think the lapse rate would be much smaller. I hope you will continue to follow the discussion at SOD site and keep making comments.
14 Al Tekhasski
ReplyDeleteYour comment "any gas has molecular viscosity, and without continuous source of stirring all motion will fade away and eventually come to total halt" is true for a quantity of gas in a zero gravity and using macroscopic gas flow currents as the criterion for motion. However, viscosity is a not a physical quantity, but a representation of the effect of gas diffusion in a bulk velocity gradient. The actual molecules never come to a stop (that would be the definition of cooling to absolute zero). Since the atmosphere is in gravity, the currents dying out due to viscosity would actually result in a small vertical temperature variation with height, and that would be sustained by diffusion, which would go on without external drivers. In an atmosphere several km tall, this diffusion would cause the so called adiabatic lapse rate to form and be maintained unless the NET radiation flux level were much large than diffusion and any convection. The convection does not even have to come from heating the lower level, but can come from any source of external non uniform (with area) heating and radiation to space.
Leonard,
ReplyDeleteThanks - yes, I've been pretty much in agreement with you, at least on the second thread. I hope I didn't give too contrary an impression. The difference has been my push to consider the energy/entropy balance. I think in the absence of a through flux, which is the case with your shell, then it falls entirely to the heat pumping from motion to counter the modes of conduction down the gradient. The heat pump drains KE, which must be restored by a heat engine. The heat pump needed for the lapse rate maintenance and the heat engine are comparable mechanisms. When you look at the thermodynamics, I just can't see a lot of energy available for the heat engine.
Incidentally, I've been talking about Rosseland mode transfer down the lap[se rate gradient. I've just realised that my simplified equation is just Eq 1 in Ramanathan and Coakley(1978).
Leonard, the original context of Nick's post was about "vertical component". He wrote: "Any gas in motion in a gravity field will have a vertical component to the motion. When it moves down or up, it is compressed or rarefied.". Therefore, is must be quite obvious that my comment was about _macroscopic_ motion of gas, and not about Maxwell-Boltzman molecular motion.
ReplyDeleteWhile we are in agreement that NON_UNIFORM heating (and planet rotation BTW) would cause atmospheric stirring and result in polytropic lapse rate, on a hypothetical planet with IR-inert atmosphere without sources of [macroscopic] stirring all macroscopic motion will die out due to molecular viscosity. As result, all temperature inhomogeneities will eventually diffuse into a constant temperature distribution, with zero gradients in any direction. There will be no lapse rate without macroscopic external drivers.
This meme is still going on at Climate etc. - the only point where I disagree is in your electrical analogies, you should have used a battery which was a current source, not a voltage source. Then, for the non-GHE case, the resistance is zero while for the GHE case, the resistance for the flow is finite resulting in a potential gradient that develops across the resistance to ensure that the flow happens.
ReplyDeleteRB,
ReplyDeleteWell, yes, the suggestion that potential can build up in a potential-driven flow may seem odd. I didn't intend either a voltage or current source, but mixed. If you add resistance into a circuit, the voltage on the side the current enters that resistance will usually rise. At that point, the resistance generally is getting the current from a finite impedance source.
Nick,
ReplyDeleteNot sure what you mean - but imagine a simple semiconductor-based current source like this: you can generate a current that is referenced to a constant voltage such as a bandgap voltage reference. Then you have to mirror this current to an open collector (bipolar) or open drain (MOSFET device). This is a current source which will maintain a fixed current up to a certain limit based on the supply voltage and has a high output impedance as a result.
For earth, the sun is the current source and the atmospheric discharge path is the resistance as you identify.
RB,
ReplyDeleteActually, I've realised the problem with my original statement - I should have said potential gradient, or perhaps differential, rather than just potential. If the same current has to get through and the resistance rises, the voltage difference has to rise.
I agree that the solar heat flux is best seen as a current source.
The lapse rate exists because the heat removed from the surface by covection and evaporation is lost by radiation at each level, so the tewmperature of the atmosphere falls. It is influenced by human sctivity and it is far more umportant than any effect of absorption of infra red by trace gases
ReplyDeleteThe surface temperature of the Solar system’s inner planets is determined totally and absolutely by three factors, one is the distance of the planet out from the sun. The second is the planet’s surface geology and the third is the quantity of greenhouse gasses in the planet’s atmosphere. For a comprehensive explanation See B at http://bit.ly/kDjJ2M
ReplyDeleteI might explain myself. For a long period I raced sailplanes. I also did daily metrological forecasts for regional and national gliding competitions. So I understand the air. My father’s concept, the KEYLINE System of sustainable agriculture, is now taught in collages all over the world.
Almost a quarter of a century ago I combined the two disciplines and produced the concept of using agricultural soils to combat global warming. I wrote papers and I lectured both here in Australia and in the US on the concept. It has subsequently been adopted, or being considered to be adored as a solution to global warming in many countries. You can see my profile on Wikipedia.
Thanks Allan,
ReplyDeleteInteresting sites. I agree about the sun, although of course the Earth;s oceans are a special factor too (and clouds).
"he important thing to note is that the dry adiabat has nothing to do with IR properties. It only requires gravity and motion."
ReplyDeleteI think this comment needs more consideration. For instance this paper by Verkley and Gerkema is relevant:
“”A column of dry air in hydrostatic equilibrium ….. bounded by two fixed values of the pressure, and the question is asked, what vertical temperature profile maximizes the total entropy of the column? Using an elementary variational calculation, it is shown how the result depends on what is kept fixed in the maximization process. If one assumes that there is no net heat exchange between the column and its surroundings—implying that the vertical integral of the absolute temperature remains constant—an ISOTHERMAL profile is obtained in accordance with classical thermodynamics and the kinetic theory of gases”
http://www.nioz.nl/public/fys/staff/theo_gerkema/jas04.pdf
If that would not be the case, it would be possible to build a machine that makes mechanical energy from a single source of heat. This goes against the 2nd law of thermodynamics.
The natural intuition that the temperature must be warmer at the bottom, because molecules gain energy, when falling, is wrong because it’s exactly compensated by another phenomenon: Those molecules that have little energy cannot go up as well as those with more energy.
This is not to deny that the sudden imposition of a gravitational field on a column of gas would
indeed set up a temperature gradient. It would – but it wouldn’t persist. It would
quickly be homogenized and the column would become isothermal.
So if a column of gas behaves this way would a GH free atmosphere behave the same way too? I think it might. Without the driving force of IR radiation from the upper reaches of the atmosphere there would be very little net heat flux in our column of air. There would be no re-radiation of course and very little convection. A GH free atmosphere would be very different from our present one and approximately much more closely to an isothermal state.
Tempterrain,
ReplyDeleteAs I've mentioned here, it takes energy to maintain the dry adiabat. Not necessarily a lot - it comes from the motion of the air, and something has to keep stirring it. There is an active pump, which pulls heat down against the temp gradient. That's what keeps it from reverting to the max entropy state.
The energy of course does ultimately come from radiation. I did an entropy budget here. Because the (conserved) energy is imported hot and exported cold, there's an export of entropy, which prevents the max state being reached.
There is an interaction with thermal radiation. This operates to move to the max entropy state, and so the heat pump has to wark against it. I suspect that this radiation leak, rather than the LH leak implied in the moist adiabat, is the main reason why the dry adiabat gradeient is not normally reached.
Thanks for this great article !
ReplyDeleteI think the difference between the Earth and Venus is that on Venus the IR "fog" clears at about 45 km above the surface rather than about 5.2 km above the surface of Earth - hence the large surface temperatures.
Thanks Clive, and sorry about the slight moderation delay - I had set moderation for older posts.
DeleteYou can see that at various levels. One obvious difference is that there is just a lot more GHG to block IR. But yes, the lapse rate over more altitude helps too.
An interesting thought experiment is, what would happen if you added 90 atmospheres of N2 to the Earth, keeping the same GHG. The lapse rate effect would be much as on Venus. The fog would clear lower, but still at a higher level than the present Earth. Would it get a lot warmer?
I think it would get warmer, but not a lot. The reason is that there is a spectral window radiating direct to space. The N2 wouldn't affect that. Much warming and there would be huge heat loss through that window.
Just to add from what I previously wrote.
ReplyDeleteI'm really questioning your statement "The important thing to note is that the dry adiabat has nothing to do with IR properties. It only requires gravity and motion. "
I don't think gravity is the explanation for the reasons above. Motion? That largely arises because of the IR properties of the atmosphere causing convection.
Temp,
ReplyDeleteGravity is certainly involved, the lapse rate is proportional to g. Motion results from any thermal inhomogeneity. Whenever air can transport heat from a hot place to a cool place, it will; there's energy to drive it. "Hot" here has to be seen in terms of potential temperature - allowing for P-V effect.
I think IR actually damps convection. Convective stability is proportional to the extent to which the gradient falls short of the adiabatic lapse rate. IR helps reduce the gradient by moving heat down it.
ps sorry about the delay. Old posts have comments on moderation to reduce spam. Since I get few for that time, I don't check as often as I should.
I Dispute the statement lapse rate depends on gravity and motion (okay I am going against accepted physics here) but I do not believe motion is necessary, lapse is due to gravity alone and I believe in a static stable atmosphere we would still have lapse rate, I believe that transfer of heat between warm high pressure and cold low pressure does not happen because they have similar entropy.
ReplyDeleteI wish it was possible to prove this with an experiment, like a vertical insulated tube several hundred meters high (no motion) with thermometers top and bottom, I am sure we would still detect a difference.
Well, yes, established physics is against you. The blogosphere is kinder. Here is a naive description of an experiment which claims to be what you wish for.
DeleteI've posted more on this, most recently here. A temperature gradient necessarily generates entropy. It takes energy to move that to somewhere else (heat pump). That is what air motion provides.
none of their lying will ever change the fact that venus radiates into interplanetary space more energy than it receives from the sun
ReplyDeleteWell, so does the Earth. But hoiw much?
DeleteBringing up a really old thread but I hope someone sees this and can answer. (Engineering background looking into some of this climate stuff)
ReplyDeleteQuestion to follow a few initial points to shape it:
"At a uniform surface, the temperature required to do this is about 255K. This is much less than the temperatures we have, and the difference of about 33K is ascribed to the greenhouse effect."
I take it there is no dispute that this is an average temperature and that daily minima and maxima would be slight extremes on either side of this as observed on the moon.
"It only requires gravity and motion. The N2 atmosphere will have that g/cp gradient. But it will start from the surface temperature fixed by the IR balance, and get colder as you go up."
I then take it the argument goes that the lapse rate is set by the average black body temperature and therefore, necessarily, average global temperatures would be much colder than if there was a greenhouse effect. But there is absolutely no doubt that the transparent gas is able to absorb (and vice versa during the night) heat from the surface, conduct and convect.
The question, very crude I know, and I assume i must have grossly misunderstood somewhere along the line (if someone can correct me I would greatly appreciate it).
God gives earth (on whatever day) an atmosphere of cold pure nitrogen. The energy from the sun heats up the surface of the earth. For a while the earth receives more energy than it emits as the surface interacts with the cold nitrogen. Eventually, the heating of the nitrogen will stop and an equilibrium established so that energy in and energy out are equal. The question is where is that equilibrium?
GHG proponents would say that equilibrium would reside where the lapse rate "sets" the atmospheric temperature equal to the average blackbody temperature at the surface. I pose that the surface is able to transmit energy to the atmosphere much more easily during the day cycle than the atmosphere is able to give up its energy to the surface during the night cycle, thus the equilibrium would be set by this balance.
All energy at the surface can be easily be transferred to molecules in the atmosphere as a whole, but the reverse is not true due to the huge, buoyancy and convection effects on the molecules that hold the energy. Is it possible that the equilibrium, and therefore the average temperature, would actually reside closer to the maximum black body radiation temperature than the average? And that temperature is actually determined by the mass and heat capacity of the atmosphere than by any radiative effects? Conduction and convection are king and that radiation serves mainly to determine the source of emission?
As i understand, there is a consensus that a transparent atmosphere's temperature would tend to the temperature of the average blackbody temperature. This seems like a gross oversimplification to me....
I'd also like to add my question also applies to the significant temperature difference between the equator and the poles and the atmospheres ability to "give back" energy, rather than a day/night cycle.
ReplyDeleteThales
Delete"that daily minima and maxima would be slight extremes"
The assumption of "uniform surface" means that it is at the same temperature everywhere, at all times. It's just to get a base level. It isn't developed very much further, because it would belabor scenarios that have never happened.
"GHG proponents would say that equilibrium would reside where the lapse rate "sets" the atmospheric temperature equal to the average blackbody temperature at the surface. I pose that the surface is able to transmit energy to the atmosphere much more easily during the day cycle than the atmosphere is able to give up its energy to the surface during the night cycle, thus the equilibrium would be set by this balance. "
Equilibrium means when all the kinetics have settled. Heat transfer to a N atmosphere is not very important; the heat capacity of the gas is low, though still affects a diurnal cycle, and there is nowhere for the heat to go.
"As I understand, there is a consensus that a transparent atmosphere's temperature would tend to the temperature of the average blackbody temperature."
That can be worked out. It's a matter of T^4 averaging. Radiation from the surface is the only way heat gets out, and long term it has to balance what comes in. The atmosphere can smooth the diurnal cycle a bit, but can't disrupt that basic calc. Lattitude difference puts more strain on the T^4 calc, but again doesn't affect the energy balance principle.
On Venus, the temperature is fairly uniform. Rotation is slow and winds are fast. Earth without GHG would also be windier. Equator->Pole is the big heat engine source, and GHG radiation is a leak that lowers its efficiency.
Many thanks for the response Nick.
DeleteI perhaps have not worded my question particularly well. What I'm saying is that I have absolutely no dispute that ultimately radiation in = radiation out. But this doesn't necessarily tell us much about the total energy stock of the system at equilibrium.
"The assumption of "uniform surface" means that it is at the same temperature everywhere, at all times. It's just to get a base level. It isn't developed very much further, because it would belabor scenarios that have never happened."
My point is that the surface of the earth is not a uniform surface and does at points reach temperatures much greater than any "base level" GHG affect or not. I wonder if these "hot spots" can drive more energy into the gas than would have been possible if the temperature was uniform (certainly true locally). As you say, there is nowhere for this energy to escape to until it interacts with the surface again. Perhaps I'm getting confused by thinking in planetary terms but I can seen a higher equilibrium temperature could be driven by these surface to atmosphere energy store interactions without disrupting the in/out radiation balance. What am I missing?
Thales,
ReplyDelete"ultimately radiation in = radiation out. But this doesn't necessarily tell us much about the total energy stock of the system at equilibrium."
Really, it does. Radiation out is determined by surface temperature, and by impedance (GHG). So radiation in implies temperature if with no GHG there is no impedance. And temperature is usually a measure of energy stock.
At steady state, energy stores don't matter. It's just flux balance. Non-uniform temperature complicates the average, and can drive a heat engine (winds, and currents if there is ocean). That evens up the temperature somewhat.
"So radiation in implies temperature if with no GHG there is no impedance. "
DeleteBit of a tautology no? This is only true if the application of the Stefan-Boltzmann equation to a planetary body with transparent atmosphere is true. This application to establish non GHG temperature is exactly what I'm querying.
I'm still not sure I'm phrasing this very well, one more way i can try an put this. Let's say the equator receives ~1300 W/m2 radiation, a more extreme latitude receives ~300W/m2. If a perfect blackbody, it receives ~1300W/m2 radiation and emits it straight back into space once at temperature, as does the more extreme latitude. I am querying why it is not possible that instead of the equator radiating this ~1300W/m2 back into space, the atmosphere carries this energy to a more extreme latitude and emit it there increasing the ~300W/m2 output from the latitude to say 400W/m2, obviously increasing the temperature in the process. Whether the radiation at the equator is emitted directly to space or transferred to the atmosphere makes no difference to the temperature there locally, but the transport of this energy certainly makes a difference globally, but at no point has the in/out balance been violated, it has just been "impeded" and redistributed, increasing the observed temperature but not the radiation out, just as is the case with the back radiation account of things.
By implication I'm then suggesting that the GHG effect is so completely unquantified to the point that the sign in front if the effect could even be open for speculation. Given the position of past and present science I have to assume I'm wrong, I'm just struggling to see why.
Delete"This is only true if the application of the Stefan-Boltzmann equation to a planetary body..."
DeleteI'm thinking of S-B locally applied. That's why I referred to T^4 averaging. Integrating W/m2 over area.
" I am querying why it is not possible that instead of the equator radiating this ~1300W/m2 back into space, the atmosphere carries this energy to a more extreme latitude and emit it there increasing the ~300W/m2 output from the latitude to say 400W/m2, obviously increasing the temperature in the process."
Certainly that happens. To emit 400 W/m2, the temperature must be raised. The total outgoing is determined by the global average T^4, modified by varying emissivity. I'm referring to the T that exists, however it got that way. The uniform surface assumption makes sense in the limit of that redistributive effect. Very strong winds.
There is a maximum entropy principle here, which I have described here. The Earth has to export the entropy that it creates. Redistributing and evening up temp increases the entropy exported. That reflects the heat engine action involved in the redistribution.
"Certainly that happens. To emit 400 W/m2, the temperature must be raised."
DeleteSo then do we agree that the radiation is not emitted at the equator but the temperature at the equator says the same?
"The total outgoing is determined by the global average T^4, modified by varying emissivity. I'm referring to the T that exists, however it got that way."
Which then means this is contradictory as this assumes that all T's are blackbody emitters, when I'm saying the T at the equator is not emitting IR energy to space but conducting to the atmosphere?
"So then do we agree"
DeleteNo, I think we are at cross purposes. Everywhere, emission is according to SB, with emissivity. Determined by T. There is also a circulation, driven by T, which moves heat within the atmosphere. This doesn't affect the application of S-B; it affects the equilibrium T to which it is applied.
I think you are confusing what responds to T, and how T got to be what it is. You can calculate a T for black-body planet without atmosphere. With no-GHG atmosphere but black emissivity, there is redistribution. In fact, the heat radiated is proportional to T^4, locally. The space integral of T^4 is fixed by total incoming heat flux. Redistribution won't change it.
Of course, right you are. An object can't forgo IR emission without giving up temperature. What an incredibly silly thing to say.
DeleteSomething still doesn't sit quite right with me on either side of these "arguements" but I'll clearly need to brush up on my electromagnetic radiation before i can ever hope to reconcile anything.
Many thanks Nick,